Waveguide Micro-probes for Optical Control of Excitable Cells
- Category: Medical Electronics, MEMS & BioMEMS, Optics & Photonics
- Tags: clifton fonstad
Optogenetics is the safe, effective delivery of light-gated membrane proteins to neurons and other excitable cells (e.g., muscle, immune cells, pancreatic cells, etc.) in an enduring fashion, thus making the cells permanently sensitive to being activated or silenced by millisecond-timescale pulses of blue and yellow light, respectively[1]. This ability to modulate neural activity with a temporal precision that approaches that of the neural code itself holds great promise for human health and for studies of brain function and interconnectivity.
We have developed multiple light guide microstructures produced using standard microfabrication techniques to deliver light to activate and silence neural target regions along their length as desired[2]. Each probe is a 100- to 150-micron-wide insertable micro-structure with many miniature lightguides running in parallel and delivering light to many points along the axis of insertion (Figure 1a). Such a design maximizes the flexibility and power of optical neural control while minimizing tissue damage. We have recently created 2-D arrays of such probes (Figure 1b) so multiple colors of light can be delivered to 3-dimensional patterns in the brain, at a resolution of tens to hundreds of microns, thus furthering the causal analysis of complex neural circuits and dynamics[3].
The initial light-guide structures have been fabricated from silicon oxynitride clad with silicon dioxide and tests show excellent transmission of light with no visible loss in the taper and bend regions of the patterns [2]. Significantly, the novel 90˚ bend invented to direct light laterally out the side of the narrow probe (Figure 1c) functions as designed[2]. The optical sources for initial tests with the probe are independent laser modules coupled to one end of a fiber-optic ribbon cable. The other end of the ribbon cable is butt-coupled to the inputs of the probe via a standard fiber-optic connector ferrule. This allows for increased modularity and control in initial probe testing.
We are now utilizing transgenic mice, which express optogenetic activators and silencers in cortical pyramidal neurons, to demonstrate optogenetic control of neural circuits in a fashion appropriate for in vivo circuit mapping or brain machine interface prototyping. Our goal is to explore the degree to which this technology can be used to functionally map neural network connectivity over large, multi-region circuits in the brain, and ultimately to enable a new generation of neural control prosthetics.
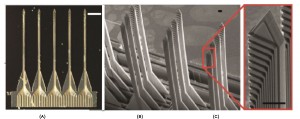
Figure 1: (a) A photomicrograph of a linear array of five waveguide probes, each which has nine guides. (b) An SEM micrograph of a 2-D probe array assembled from four linear arrays having five waveguide probes, each of which has twelve guides. (c) A zoomed-in view of the 2-D array in Figure 1b showing the 45˚ mirrors and output ports. The scale bars are all 100 µm long.
- X. Han and E. S. Boyden, “Multiple-color optical activation, silencing, and desynchronization of neural activity, with single-spike temporal resolution,” PLoS ONE, vol. 2, no. 3, Mar. 2007, p. e299. [↩]
- A. N. Zorzos, E. S. Boyden, and C. G. Fonstad, “A multi-waveguide Implantable probe for light delivery to distributed brain targets,” Optics Letters vol. 35, no. 12, Dec. 2010, pp. 4133-4135. [↩] [↩]
- A. N. Zorzos, J. Scholvin, E. S. Boyden, and C. G. Fonstad, “Three-dimensional multi-waveguide probe array for light delivery to distributed brain circuits,” Optics Letters, vol. 37, no. 23, Dec. 2012, pp. 4841-4843. [↩]