- Authors: S. Anand, A. Paxson, R. Dhiman, J. D. Smith, K. K. Varanasi
- Sponsorship: MIT
Despite years of advancement in making energy systems more efficient, the predominant mode of condensation seen in large-scale industrial processes is still filmwise condensation. Replacing the filmwise condensation mode with dropwise condensation promises large improvements in heat transfer efficiency that will reduce the cost in material, water consumption, and size of the systems. In this regard, the use of superhydrophobic surfaces fabricated by texturing surfaces with nano/microstructures has been shown to lead decreases in contact line pinning of millimetric drops, resulting in fast shedding. However, these useful properties are lost during condensation as droplets that nucleate within the texture grow larger by virtue of condensation while still adhering to the surface. This Wenzel pinning can, however, be eliminated by introduction of a lubricant[][][][]. Recently we have shown that liquid-impregnated surfaces can overcome many limitations of conventional superhydrophobic surfaces during condensation[]. Here we show aspects related to condensation on liquid surfaces and how relations among the surface tension of the encapsulating liquid and the condensing liquid determine the condensation and subsequent shedding behavior for condensing droplets (Figure 1). The characteristics of condensed droplet behavior on these surfaces are compared with that on conventional un-impregnated superhydrophobic surfaces (Figure 2) to show how the use of lubricant impregnated surfaces may lead to large enhancement in heat transfer and energy efficiencies ((Anand, S.; Paxson, A. T.; Dhiman, R.; Smith, J. D.; Varanasi, K. K., Enhanced Condensation on Lubricant-Impregnated Nanotextured Surfaces. ACS Nano 2012, 6, (11), pp. 10122-10129.)).
-
-
Figure 1: Plot comparing variation of surface area fraction covered by condensed water droplets versus time on surfaces impregnated with Krytox (Sow > 0, solid squares) and BMIm (Sow < 0, open diamonds). The ESEM experiments were conducted under identical conditions (pressure 800 Pa, substrate temperature ~3.6 oC, beam voltage 25 kV, and beam current 1.7 nA). In the analysis, t = 0 s is defined as the first frame in which water drops can be identified
-
-
Figure 2: Comparison of condensation on hierarchical superhydrophobic and ionic liquid-impregnated surfaces with identical texture at macroscales. (a) Representative image of condensation on superhydrophobic surface comprising nanograss microposts; large pinned droplets are seen be- fore shedding from the surface. (b) Representative image of condensation on nanograss microposts impregnated with ionic liquid. Both small and large droplets are highly mobile; colored circles are provided as visual guides for depicting droplet motion. (c) Median speed of droplets as a function of drop diameter for unimpregnated (black circles) and impregnated surfaces (orange circles). The data are plotted on a mixed linear/logarithmic scale in order to capture both the zero value and substantial range of magnitudes in droplet speed.
- Authors: G. Azimi, H. Kwon, A. T. Paxson, K. K. Varanasi
- Sponsorship: NSF, Dupont-MIT Alliance, MIT Energy Initiative, DARPA
Hydrophobic materials that are robust to harsh environments are needed in a broad range of applications[][][]. Although durable materials such as metals and ceramics, which are generally hydrophilic, can be rendered hydrophobic by polymeric modifiers[], these materials deteriorate in harsh environments. Here we show that a class of ceramics comprising the entire lanthanide oxide series, ranging from ceria to lutecia, is intrinsically hydrophobic (Figure 1)[]. We attribute their hydrophobicity to their unique electronic structure, which inhibits hydrogen bonding with interfacial water molecules. We also show with surface-energy measurements that polar interactions are minimized at these surfaces and with FTIR/GATR that interfacial water molecules are oriented in the hydrophobic hydration structure. Moreover, we demonstrate that these ceramic materials promote dropwise condensation, repel impinging water droplets, and sustain hydrophobicity even after exposure to harsh environments[]. These ceramics can also be used to fabricate superhydrophobic surfaces using various techniques. As an example, we fabricated superhydrophobic surfaces by sputtering a thin layer (~200-350 nm) of ceria onto nanograss-covered silicon microposts (Figure 2a). Water droplets display high contact angles (Figure 2b) and exhibit extreme mobility due to low contact angle hysteresis (<10°) on these surfaces. In addition, impinging water droplets completely bounce off the surface, leaving it dry (Figure 2c). Hence, we envision that this class of robust hydrophobic materials will have far-reaching technological potential in various industrial applications, where water repellency and dropwise condensation are desirable.
-
-
Figure 1: Photographs of the sintered rare-earth oxide pellets (cerium oxide through lutetium oxide). All the pellets are hydrophobic: water contact angles range between 100° and 115°. The image at the center is a goniometer snapshot of a water droplet beading up on a smooth neodymium oxide surface, as a model sample of the entire REO series; scale bar is 1 mm.
-
-
Figure 2: Superhydrophobicity and water repellency of rare-earth oxide coated textured surfaces. a) SEM of nanograss-covered microposts (side ~ 10 μm, inter-post spacing ~ 5 μm); scale bar is 10 μm; b) Photographs of water droplets on the ceria sputtered nanograss micropost surface (θ =160° ± 2°); scale bar is 2.5 mm.; c) High-speed photography images of a water droplet bouncing off the surface (impact velocity ~ 1.6 m/s); scale bar is 2.5 mm.
- Authors: A. T. Paxson, K. K. Varanasi
- Sponsorship: Department of Mechanical Engineering, Massachusetts Institute of Technology
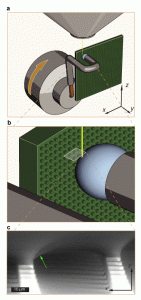
Figure 1: ESEM fixture and micrograph. (a) Experimental apparatus within an ESEM chamber. A water droplet is held against a vertically oriented superhydrophobic micropillar surface by a copper wire. Water drop is cooled by a Peltier device and can be swept across the surface (x-direction) by rotating the ESEM stage about the y-axis. (b) Illustration of electron beam imaging area with respect to droplet contact line. (c) Single micrograph from video of water droplet receding along superhydrophobic micropillars in positive x-direction.
We report a technique for observing the dynamic behavior of a liquid contact line on superhydrophobic surfaces at high magnification using environmental scanning electron microscopy (ESEM). Superhydrophobic surfaces utilize a combination of low surface energy and high roughness that lead to a Cassie-Baxter (CB) wetting state, in which the drop sits atop roughness features on a composite interface[][][]. Adhesion is substantially reduced in the CB state, but it is not altogether eliminated. In fact, the adhesion of CB drops represents an almost ideal case of strong dilute defects for investigating the effects of contact line pinning[]. When a CB drop is moved across a surface, the deformations due to pinning are localized at the receding region; as the contact line is displaced, it is hypothesized that micro-capillary bridges are formed at each pinning site[][][]. The behavior of these capillary bridges ultimately determine the adhesion of the entire drop. In the current study, we are able to capture micron-scale images of capillary bridges formed as the contact line recedes across a superhydrophobic micropillar surface (Fig. 1) and measure the local receding contact angle. We find that it is equivalent to the macroscale receding contact angle observed on a chemically equivalent smooth surface and satisfies the Gibbs depinning criterion[]. On a dual-hierarchy surface consisting of roughened micropillars, the capillary bridges exhibit a larger local receding angle. We attribute these effects to a self-similar depinning mechanism that depends on the pinned fraction of the contact line at each roughness hierarchy. We develop a model to predict adhesion force of a macroscopic drop and verify this mechanism with adhesion measurements. This mechanism helps to explain the necessity for multiple length scales exhibited by lotus leaves and other superhydrophobic surfaces and also helps explain how complicated pillar geometries lead to increased pinning.