Non-resonantly Pumped High-quality-factor Lasers
Lasing Solar Concentrators are expected to have record efficiency in converting light to electricity. They are basically solar-pumped lasers, which, due to the low intensity of the solar flux, need to possess a high-quality factor (high Q). High-Q lasers are also a key feature in on-chip optical computing. Currently, high-Q lasers can only be resonantly pumped by a second high-Q laser, resulting in oscillations in the lasing output [1]. In this work, we show a new pumping technique for high-Q lasers that is independent of the coherence of the pump source. This technique is based on cascaded energy transfer that resembles adiabatic transfer from incoherent to coherent light. Our work opens the way to solar-pumped lasers and solves a major bottleneck towards the realization of optical computing.
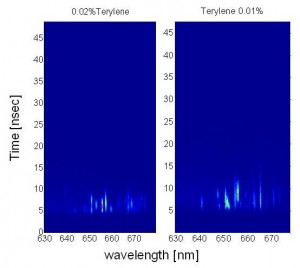
Figure 2: Typical streak camera results, showing extension of photon lifetime due to reduction in Terrylene concentration.
In the last few months, we have optimized the cascaded energy-transfer to yield record Q-factor for organic lasers (Q=10000). The energy-transfer starts with AlQ3 molecules that absorb the UV pump light and transfer energy to DCJTB molecules, which then transfer the energy to Terrylene, which in turn lases at about 680-nm wavelength. The concentration of the Terrylene is about 0.001%. Lasing at such a low concentration (and thus highly transparent media), enables the propagation of the lasing photons for long distances in the cavity, which is reflected at the high Q-factor that we observed. Figure 1 shows a typical power spectrum measurement, with FWHM at the measurement limit of 0.07 nm.
The observed Q-factor is [latex]Q=\theta\tau=\frac{\theta}{\Delta\theta}=\frac{\lambda}{\Delta\lambda}\sim\frac{700}{0.07}=10000[/latex] and is limited by the measurement limit of the spectrum analyzer. Our current goal is to measure the real Q-factor at this optimized configuration by measuring the lifetime of the photon in the cavity. For this measurement we use a streak camera. This measurement allows us to differentiate between DCJTB and Terrylene emissions. We examine whether the Q-factor of the Terrylene is significantly longer than that of the DCJTB. Also we the measure Q-factor-dependence with the concentration (absorption) of Terrylene. Preliminary measurements of photon lifetime vs. concentration of Terrylene are shown in Figure 2. These results suggest a Q-factor greater than 500000.
References
- T. Carmon, and K.J.Vahala, “Modal spectroscopy of optoexcited vibrations of a micron-scale on-chip resonator at greater than 1 GHz frequency,” Phys. Rev. Lett. 98, 123901 (2007). [↩]