Direct Seawater Desalination by Ion-concentration Polarization
The shortage of fresh water is a serious global problem, and an energy-efficient desalination strategy can provide a substantial solution for the water-crisis [1]. Current desalination methods utilizing reverse-osmosis and electrodialysis mechanisms require high power consumption or large-scale infrastructures, which are not suitable for resource-limited settings. This work elucidates a novel desalination process utilizing ion-concentration polarization (ICP) [2].
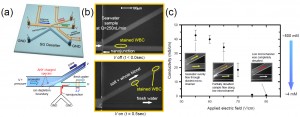
Figure 1: Schematic diagram of (a) micro/nanofluidic desalination system with embedded microelectrode for measuring conductivity and (b) electrokinetic desalination operations associated with external pressure field. (c) The conductivity of desalted stream in experiments with seawater sample.
Often called ion depletion or enrichment, ICP occurs due to the mismatch of the charge carriers at the nanoporous membrane. Once ICP is triggered, the concentrations of both cations and anions decrease on the anodic side of the membrane (ion depletion) and increase on the cathodic side (ion enrichment) [3]. With a strong ICP, both anions and cations are depleted near the nanojunctions. If ICP is combined with a pressure-driven flow, a steady-state depletion zone is obtained using the device depicted in Figure 1(a). In the experiments done with seawater obtained from Crane Beach, MA in Figure 1(b), the depletion zone was formed to divert charged ions (represented by dyes) into the “salted” stream. It was also shown the ICP layer acts as a virtual barrier for all charged particles, including most solid particles and biomolecules found in water. Therefore, both small salt ions and large microorganisms can be removed from the “desalted” stream, significantly reducing membrane fouling. Figure 1(c) shows in situ conductivity measurement of “desalted” stream. Measurements of the desalted stream indicate the seawater’s conductivity dropped from ~50mS/cm to ~0.5mS/cm, which corresponds to ~3mM salinity. This level of salinity is below the 10mM threshold required for potable water.
The steady-state current required was found to be ~30µA, equating power consumption of ~3.5Wh/L. This technology could be applied to small-scale desalination systems, possibly with the option of battery/solar cell-powered operation. The footprint of the device is small, potentially allowing ~3X104 parallelization on the wafer scale for a total throughput of ~300mL/min.
References
- M. A. Shannon, P. W. Bohn, M. Elimelech, J. G. Georgiadis, B. J. Mariñas, and A. M. Mayes, “Science and technology for water purification in the coming decades,” Nature, vol. 452, p. 301, 2008. [↩]
- S. J. Kim, S. H. Ko, K. H. Kang and J. Han, “Direct seawater desalination by ion concentration polarization,” Nature Nanotechnology, vol. 5, p. 297, 2010. [↩]
- S. J. Kim, Y.-C. Wang, J. H. Lee, H. Jang and J. Han, “Concentration polarization and nonlinear electrokinetic flow near a nanofluidic channel,” Physical Review Letters, vol. 99, no. 4, p. 044501, 2007. [↩]