Continuous-flow Deformability-based Sorting of P. falciparum-infected Red Blood Cells Using a Micro-filter Array
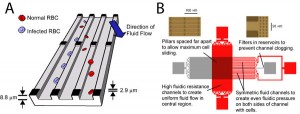
Figure 1: A. Illustration of device structure and operation. Fluid flows diagonally with respect to the slits. Infected RBCs slide along the edge of a slit, while normal RBCs follow the direction of fluid flow. B. Details regarding device architecture.
Change in cell stiffness is a characteristic of several blood cell diseases, such as sickle cell anemia [1], malaria [2], and leukemia [3]. In humans, the spleen acts like a filter to remove these more rigid cells by pushing blood through slits between endothelial cells and removing cells that cannot pass. In this work, we create a microfluidic device that mimics the architecture of the spleen to achieve continuous-flow fractionation of cells based on their rigidity. We demonstrate successful operation of this device by separating malaria-infected from normal, uninfected red blood cells (RBCs). Applications include disease diagnosis and sample preparation for downstream analysis. Figure 1 illustrates device operation and presents details regarding device design.
The device fabrication process involves the use of photo-curable polyurethane, NOA 81. The high Young’s modulus of this material enables the creation of high aspect ratio slits in an inexpensive and simple manner, as contrasted with PDMS and silicon-glass. Fabrication details have been presented elsewhere [4].
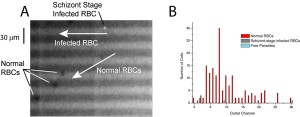
Figure 2: A. Still frame from movie. Malaria-infected RBCs stained with Hoechst dye fluoresce under UV illumination, while uninfected cells appear as shadows. B. Demonstration of device selectivity. The device was able to isolate 4 schizont-stage infected RBCs from 164 uninfectedcells.
We separated schizont-stage malaria-infected RBCs from uninfected RBCs. Figure 2A presents a snapshot from a video showing an infected cell sliding along the edge of a slit and uninfected cells following the direction of the fluid flow. Figure 2B presents a histogram showing the separation sensitivity of the device. As shown, this device was able to separate 4-infected cells from 164 uninfected cells.
Applications of this device include sample preparation for biochemical and cell culture assays. Deformability-based cell sorting can also be useful for situations when cell surface markers are not clearly identified for FACS. Lastly, the low-cost aspect of this device makes it ideal for on-site disease (e.g., malaria) screening in resource-poor settings.
References
- E. Evans, N. Mohandas, and A. Leung, “Static and dynamic rigidities of normal and sickle erythrocytes: Major influence of cell hemoglobin concentration,” J. Clin. Invest, vol. 73, pp. 477-488, 1983. [↩]
- J. P. Shelby, J. White, K. Ganesan, P. K. Rathod, and D. T. Chiu, “A microfluidic model for single-cell capillary obstruction by plasmodium falciparum-infected erythrocytes,” PNAS, vol. 100, pp. 14618-14622, 2003. [↩]
- M. J. Rosenbluth, W. A. Lam, and D. A. Fletcher, “Force microscopy of nonadherent cells: a comparison of leukemia cell deformability,” Biophysical Journal, vol. 90, pp. 2994-3003, 2006. [↩]
- H. Bow, P. Abgrall, and J. Han, “Microfabricated slits in series: A simple platform to probe differences in cell deformability,” Proceedings of MicroTAS 2008 Symposium, vol. 2, pp. 1199-1201, 2008. [↩]