Timing Performance of Superconducting Nanowire Avalanche Photodetectors
- Category: Electronic Devices, Nanotechnology, Optics & Photonics
- Tags: Faraz Najafi, Francesco Marsili, Karl Berggren
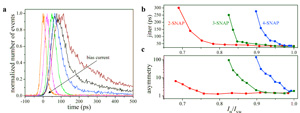
Figure 1: (a) Instrument response function (IRF) of a SNAP based on two 30-nm-wide nanowires (2-SNAP). (b) Jitter of a 2-, 3-, and 4-SNAP based on 30 nm wide nanowires as a function of the normalized bias current (IB / ISW). (c) IRF asymmetry of the same devices as in (b).
Superconducting nanowire avalanche photodetectors (SNAPs) [1] are based on a parallel architecture that performs single-photon counting with higher signal-to-noise ratio (up to a factor ~4) than traditional superconducting nanowire single-photon detectors (SNSPDs) [2] . Although the understanding of the operation mechanism of SNAPs was recently improved [3] [4] , a comprehensive study of the timing performance is still lacking. In the only study reported so far [5] , SNAPs showed significantly higher timing jitter (>250 ps FWHM) than SNSPDs (~30ps FWHM [6] ).
We report a study of the SNAP timing performance for several device architectures and bias regimes. Our main findings were: (1) the instrument response function (IRF) shifted to longer delay times when the bias current was decreased (Figure 1.a); (2) when properly biased, SNAPs achieved the same jitter as SNSPDs (Figure 1.b); and (3) the IRF became more asymmetric when the bias current approached the avalanche current (Figure 1.c).
We simulated the electro-thermal dynamics of SNAPs using the model described in [3] . While we could not explain the origin of the IRF asymmetry, the IRF shift to longer delay times was shown to be caused by a change in the electro-thermal behavior of the detectors at decreasing bias currents. We conclude that SNAPs are suitable for timing-sensitive single-photon experiments while offering a higher signal-to-noise ratio than standard SNSPDs.
- M. Ejrnaes et al., Appl. Phys. Lett. vol. 91, p. 262509, 2007. [↩]
- G. N. Gol’tsman et al., Appl. Phys. Lett. vol. 79, p. 705, 2001. [↩]
- F. Marsili et al., Appl. Phys. Lett. 98, p. 093507, 2011. [↩] [↩]
- F. Marsili et al., Nano Lett., 2011, 11 (5), pp 2048–2053. [↩]
- M. Ejrnaes et al., Appl. Phys. Lett. 95, p. 132503, 2009. [↩]
- E. A. Dauler et al., IEEE Trans. Appl. Supercond. vol. 17, p. 279, 2007. [↩]